Overcome the Challenges of Using Sub-Milliohm SMD Chip Resistors--part 1


In this article, you’ll learn:
How to treat sub-milliohm chips as a separate class of component.
Strategies to avoid pitfalls during component selection and PCB layout design.
Uncover opportunities to quantify and minimize error and variation at every stage.
The simplest and most cost-effective way of converting a measured current to a voltage signal is to use a low-ohmic-value current-sense resistor. The upswing in products containing batteries, motors, or actuators that call for current monitoring or control has led to huge market growth for current-sense chip resistors with values below one ohm over the last two decades.
More recently, though, driven by power-efficiency demands and enabled by low-noise voltage-sense amplifiers, the value range has extended downward from milliohms to hundreds of microhms. Such low ohmic values present challenges to the user at many stages in their design and manufacturing processes.
This two-part series considers the nature of these challenges and suggests strategies to overcome them at various stages, including component selection, PCB layout design, verification of the ohmic value of unmounted components, and critical assembly processes. Each stage features potential pitfalls but also opportunities to quantify and minimize error and variation.
Although sub-milliohm chip resistors are still just chip resistors, ideal design strategies treat them as a separate class of component, embracing considerations and techniques that enable their successful use.
Component Selection
Termination Styles
When restricting the temperature sensitivity of a resistor-based current-sensing circuit, it’s well-known that the total terminal resistance from copper, common to both the current-carrying circuit and the voltage-sense circuit, must be restricted as well. That’s because this element of the total measured resistance has a temperature coefficient of resistance (TCR) of +3900 ppm/°C, which contributes to the total TCR in proportion to the resistance ratio.
For example, a total terminal resistance of 100 µΩ, i.e., 50 µΩ at each end, for a 1-mΩ resistor contributes 100 µΩ/1000 µΩ × 3900 ppm/°C = 390 ppm/°C to the TCR. This contrasts with the TCR of the resistance element itself, which is typically better than ±30 ppm/°C. Such separation between the current-carrying and voltage-sense circuits is referred to as a Kelvin connection, an issue that becomes clearly more important as the nominal ohmic value is reduced.
There’s a spectrum of termination styles to address this problem. The most common is the Kelvin connectable two-terminal resistor (Fig. 1a). While this is often the lowest-cost option, it places the onus on the PCB designer to realize a Kelvin connection in the PCB track layout (later we’ll look in detail at how this may best be achieved). Such a component must have a low termination resistance since the Kelvin connection strategy necessarily ends at the surface of the termination.
(1) A Kelvin connection, or separation between the current-carrying and voltage-sense circuit, becomes increasingly important as nominal ohmic value is reduced. The most common option is a Kelvin connectable two-terminal style. (a) There’s also an intermediate option, a semi-Kelvin four-terminal style (b), and a true Kelvin four-terminal style.
Figure 1b shows an intermediate component type where four solder terminals are provided, but the separation of circuits doesn’t extend all the way into the resistor element. Figure 1c shows a true Kelvin format where there’s no current-carrying termination whatsoever within the voltage-sense circuit. The latter two types offer error-proof PCB layout design and the lowest achievable magnitude of TCR, but generally it comes at a higher cost.
Element Materials
Low-value resistors can be made from both thick- and thin-film materials, but the lowest values available in these technologies are in the multiple-milliohm range. Both types are relatively susceptible to damage from high-current surges. In the case of thick-film technology, the lowest values are associated with high TCR values of several hundred ppm/°C and thus are suited only to low precision use.
For these reasons, most current-sense chip resistors are based on a bulk metal element. This may be either a foil supported on a substrate or a self-supporting metal element. Though the former option allows for the use of thin metal layers to achieve higher values, the latter lends itself to sub-milliohm values.
A range of alloys, each with differing resistivities, are selected by device designers to provide the required ohmic value within the dimensional constraints of the product. From the point of view of the user, the material choice is often unimportant, but there are two exceptions: one is the control of thermally generated errors, and the other is application for non-dc circuits.
A copper-terminated metal-element chip resistor contains at least two boundaries between dissimilar metals. These act as thermocouples and generate a thermoelectric voltage in the presence of a temperature gradient. Furthermore, they’re connected in series, and because of the component’s symmetry, they’re of opposite polarity when the resistor element itself is the main heat source. As a result, if the temperature distribution across the chip resistor is symmetrical, any generated thermoelectric voltages will be cancelled out.
Figure 2a illustrates this balanced state in which the thermal voltages V1 and V2 are equal. Figures 2b and 2c show an example of imbalance due to the external influence of a heat source and a heatsink, respectively. This would lead to a finite value of V1 − V2, which would sum with the measured sense voltage and create a source of error.
(2) Shown is a balanced state in which the thermal voltages V1 and V2 are equal (a), and examples of imbalance due to the external influence of a heat source (b) and a heatsink (c).
In many designs, it’s simply not possible to guarantee thermal symmetry under all operating conditions. In such a case, a chosen part should employ a resistance alloy with a low thermoelectric voltage against copper. These alloys contain manganese in a copper nickel alloy in which the proportion of copper exceeds 80%. The thermoelectric voltages generated across a junction with copper can be as low as 3 µV/°C, which is an order of magnitude lower than for a copper nickel alloy.
The second application-specific driver for resistance alloy selection is the need to avoid iron-bearing alloys in circuits where ac or rapid step changes in dc need to be tracked accurately, as this is not possible with ferromagnetic alloys.
Thermal Design Format
An inherent problem with resistive current sensing is heat, generated at a rate proportional to the square of the current. This may need to be restricted for one of two reasons. First, the design must reduce the effect of temperature increase on the linearity of the component, which can stem from TCR or from thermoelectric voltage errors. Second, it’s necessary to avoid overheating the resistance alloy, which can lead to irreversible ohmic-value change.
This consideration calls for careful thermal design of the assembly. It begins at the component selection stage in response to the basic decision as to where the heat generated should be dissipated; for example, in the air or in the copper PCB tracks. The answer to this depends on the overall thermal-management strategy.
A well-ventilated assembly—with either a high thermal loading already on the PCB or with temperature-sensitive components—would benefit from a resistor that dissipates heat into the air. Conversely, a PCB that’s heatsinked, or has no excess of heat generation and no temperature-sensitive parts, can employ a resistor which dissipates heat mainly to the PCB tracks.
An example of a primarily air-dissipating open-air format is shown in Figure 3a. This can sustain a temperature rise of the hotspot above the solder joints well in excess of 100°C. Its flexible nature makes it virtually immune to temperature cycling or board flex stresses on the solder joints. An example of the primarily PCB dissipating flat-chip format is shown in Figure 3b. The design benefits from its low profile and is generally the lower-cost option.
(3) Illustrated are an open-air format metal-alloy resistor (a), and a flat-chip format metal-alloy resistor (b).
Most Common Type
After considering the many options of termination style, element
material, and thermal design format, the most common type of
sub-milliohm resistor is a two-terminal metal-element chip resistor.
This resistor type will be considered hereafter in this article.
PCB Layout Design
Near-Kelvin Connection
The PCB layout design around a very-low-value resistor is critical to its performance. The most important aspect of this design is the fact that four rather than two tracks must be provided to form a Kelvin connection, even where the component itself has only two terminals.
The aim is to minimize the conductive path shared between the current path and the voltage-sensing loop (Fig. 4), which would increase both the effective ohmic value and the TCR of the mounted part. This may be achieved by connecting the voltage-sense tracks to the inner edges of the solder pads (Fig. 5).
(4) Minimizing the conductive path shared between the current path
and the voltage-sensing loop increases both the effective ohmic value
and the TCR of the mounted part.
(5) To achieve what’s illustrated in Figure 4, connect the voltage-sense tracks to the inner edges of the solder pads.
Designers also can take this a step further and split the voltage-sense pads from the current-path pads, so that the solder joints themselves are removed from the shared path, too (Fig. 6). By using this method, it’s possible to approach the accuracy obtained from a true four-terminal resistor.
(6) By splitting the voltage-sense pads from the current-path pads, the solder joints themselves also are removed from the shared path.
Furthermore, a study by Analog Devices based on TT Electronics’ ULR3 0.5-mΩ mounting-pad options has shown that a mounted value tolerance close to 1% may be achieved on a 1% tolerance component. This indicates low additional error due to mounting effects, using a centralized, isolated sense-pad design similar to that of Figure 4c.
Minimization of Sense-Loop Area
A source of error involving high currents that are ac or changing dc is due to the voltage-sensing loop linking with changing magnetic fields. This can induce a noise signal superimposed on the desired voltage sense signal.
To reduce the noise, the loop area contained within the sense resistor, the two voltage-sense tracks, and the sense circuit input should be minimized. This means keeping the sense circuitry as close as possible to the sense resistor and running the voltage-sense tracks close to each other.
A good way to keep these tracks very close is to superimpose them in different PCB layers. Where long track runs are unavoidable, it’s also possible to use periodic vias to cross over the tracks into alternate layers. On a PCB, this replicates the effect of a twisted-pair cable, which, by means of cancellation of induced voltages, allows the circuit to withstand the effect of any changing magnetic fields that have small variations across the spatial periodicity of the twisting.
Connecting Multiple Resistors in Parallel
Sometimes, designers are forced to use more than one current-sense resistor connected in parallel, either to meet a high power or surge rating, or to achieve an ohmic value lower than the minimum available. This is problematic but possible. Resistors may be connected in parallel with voltage-sense connections made to just one of the resistors, provided the track layout ensures equal distribution of current between all resistors.
For example, the position in the current trace where the resistors are placed should be well clear of bends or constrictions that could affect the distribution of current density (Fig. 7). The goal is to ensure that the total track resistance in series with each resistor should be the same (Fig. 8), so that the sensed resistor carries the required fraction of the total current.
(7) Parallel connection requires equal distribution of current.
(8) The total series track resistance should match for all resistors.
Moreover, this ensures that the proportion of the total current carried by the sensed resistor doesn’t vary with temperature. This would otherwise be the case with unequal series track resistances due to the high TCR of the copper PCB tracks.
Design for Heatsinking
A flat-chip resistor dissipates more than 80% of its heat by conduction into PCB tracks, making it important to provide sufficient copper area to act as a heatsink. Copper area is, for this purpose, defined as the total area directly surrounding the solder pads, including the first two squares of connected tracks. Figure 9 indicates the general relationship between effective power rating and PCB copper area.
(9) The general relationship between effective power rating and PCB copper area can be divided into three distinct regions.
In region (A), there’s relatively low thermal conduction through copper connected to the pads, and conduction by substrate and convection to air predominate. In region (B), the copper connected to the pads acts as a heatsink to raise the effective power rating. In region (C), further increase in copper area gives diminishing returns, as the internal thermal impedance of the chip restricts the rating.
This limiting factor of the internal thermal impedance can be lowered significantly by changing the resistor’s orientation. If the terminations are formed on the longer edges of the chip rather than the shorter edges, the solder joint width is approximately doubled and the maximum distance from film center to termination is approximately halved (Fig. 10a). TT Electronics' ULR2N and ULR3N (Fig. 10b) are examples of products that use this enhanced cooling method.
(10) The limiting factor of the internal thermal impedance may be lowered significantly by changing the orientation of the resistor. If the terminations are formed on the longer edges of the chip rather than the shorter edges, the solder joint width is approximately doubled and the maximum distance from film center to termination is approximately halved (a). TT Electronics ULR2N and ULR3N are examples of products that make use of this enhanced cooling method (b).
The resistor datasheet should contain information on the mounting conditions used to obtain the rated power. This indicates the minimum copper area that should be provided by the designer.
Summary
The growing use of sub-milliohm chip resistors for current sensing creates a spectrum of challenges for the designer and the process engineer. The component format should first be selected to support the chosen thermal-management approach, with metal-element flat-chip resistors having two terminals being the most cost-effective solution. It’s then essential to design the PCB tracks and pads to meet the needs of the Kelvin connection, heat dissipation, and avoidance of induced noise.
Part 2 of this series will address additional stages, including verification of the ohmic value of unmounted components and critical assembly processes.
- |
- +1 赞 0
- 收藏
- 评论 0
本文由咪猫转载自TT Electronics,原文标题为:Overcome the Challenges of Using Sub-Milliohm SMD Chip Resistors (Part 1),本站所有转载文章系出于传递更多信息之目的,且明确注明来源,不希望被转载的媒体或个人可与我们联系,我们将立即进行删除处理。
相关推荐
Overcome the Challenges of Using Sub-Milliohm SMD Chip Resistors--part 2
For TT Electronics’ LRMAP2512-R0005FT4, 500 µΩ ±1% in a 2512 footprint, the value of A is approximately 8.32 mm2. And for SAC305 alloy solder, the value of ρ is 1.4 x 10-7 Ωm.
设计经验 发布时间 : 2022-05-31
【经验】TT Electronics 红外光电传感器的连接和使用方法
TT Electronics旗下品牌OPTEK了一系列光电传感器的产品,以适用于不同的应用场合。本文旨在帮助那些在连接基本光电组件方面经验很少或没有经验的人,介绍红外光电传感器的连接和使用方法。基本的光电传感器通常由两个单独的部件组成:LED(又称为“发射器”),通常会发射红外光;光电晶体管(又称为“传感器”或“探测器”),它响应红外光。
设计经验 发布时间 : 2018-08-17
【经验】TT Electronics用于DC-DC转换的高隔离电压反激变压器设计思路
TT Electronics经AEC-Q200认证的HA00-10043ALFTR、HA00-14013LFTR高压隔离变压器与其他竞争解决方案相比,其饱和能力提高10%,漏电感提高22%,并且通过其现场应用工程师,TT Electronics可以进一步帮助客户选择适合高应力环境的材料。
设计经验 发布时间 : 2019-01-08
ABCIRH Connectors Halogen Free Bayonet Lock Connector Series
目录- Company Profile and Product introduction Halogen Free Connectors Information and Part Number Explanation F80 Series Contact Arrangements ABCIRH Series Planforms F80 Series Crimp Contacts Connector & Accessory Conduit Adaptor Cable Clamp Locking 90° Outlet Adaptor Cable Clamp With Grommet Non-Locking Conduit Adaptor&Cable Clamp Long Backshell, Grommet, Cable Clamp & Bushing Step Up /Down /Threaded Adaptor CMS Series Cable Clamp Telescopic Bushing/Panel Sealing Gaskets/Protective Caps Wire Seal Grommet Square Flange /Single Hole Mounting Receptacle Connector Insertion & Extraction Tools Braid Product Product Safety Information
型号- ABBET16,ABBET12,X-PCAJABB-**-E-PCA,NON-SCREENING- SB - ** - RPG,ABCIRHP06HD,ABB - **-CRA,ABB16/20KSKF80,ABCIRHP06HC,ABBH - ** - ** - JE - F80,ABCIRH06XLA,ABCIRH06XLC,ABB28NPA,ABCIRHP06GS,ABBH - ** - ** - SHE - F80,AB,ABB12/18KPKF80,ABB100KSKF80,ABBMBS035015,CMSP3057C16,ABB16NPA,ABB16SKLKF80,CMSP3057C18,CMSP3057C20,CMSP3057C24,ABB16SG,CMSP3057C22,ABBH-40-LMGA,ABB40NPA,ABB12/40KPKF80,ABB ## #GA #,ABB1210KPKF80,ABBH-18-LMGA,ABCIRHP06JE,ABB8/10KSKF80,ABB608109,ABB16S/12KPKF80,ABBPL0550A1130,ABB16/12KSKF80,ABCIR-28-SX,ABCIRH03RC,ABB-20-#GA-#M32,ABCIR-40-SX,ABB8KPKF80,ABB12/20KLKF80,ABBH-32-LMGA,ABB28NP,ABB500/250KPKF80,ABB 6T 16,ABB500KPKF80,RFI SCREENING- SB - ** - RPGS,ABB-16-SGP,ABB16/18KPKF80,ABB10SLNPA,ABCIRH00JE,ABBH - ** - ** - FM F80,ABB16NP,ABBH - ** - ** - HD - F80,CMSP3057A18,CMSP3057A16,ABB12/10KSKF80,CMSP3057A22,CMSP3057A20,ABB16S/18KSKF80,ABBMBS025015,CMSP3057A24,ABCIRH03T...M6,ABBH-**-**-SHE-F80,ABB-IT-16,E-PCA,CMSP3057C28,ABB-IT-12,ABB10SLNP,ABCIRH00FT,ABCIR-49-SX,ABBH - ** - ** - SDA*,CMSP3057C32,ABBH - ** - ** - ACA - F80,ABCIRH00FM,ABB 4 TKSK F80 P1,ABBH - ** - ** - FC - F80,ABBH - ** - ** - XLA - F80,ABCIR-16-SX,ABB16/14KSKF80,ABCIRH00HC,ABCIRH00HD,ABBH - ** - ** - LHSA - F80,ABB12/16KPKF80,CMSP3057C36,RFI SCREENING- SB - ** - FPGS,ABB16S/22KSKF80,CMSP3057C40,ABCIRH00GS,ABB-32-#GA-#M32,ABCIRH00GM,ABB8/40KPKF80,ABBTP1249,ABBH-16S-LMGA,ABB - ** - KDP F80,ABBH - ** - ** - EV - F80,ABB22NP,ABB12KLKF80,NON-SCREENING- SB - ** - FPG,ABCIRH00FC,ABB500/250KSKF80,ABB500/160KSKF8,ABB-16S-#GA-#M32,ABCIRH03A/ABCIRH03A...M6,ABCIRH00T/ABCIRH00T...M6,ABB16/14KL8F80P3,ABCIRH06RC,ABCIRH00EV,ABCIRH03XLA,ABCIRH03XLC,ABB12SG,ABB8/12KSKF80,ABCIR-36-SX,ABCIRHP08FC,ABB500/350KPKF80,ABB0KPKF80,ABBMBS050015,ABB16S/22KPKF80,ABB500/250KPKF8,ABB12/48KLKF80P3,ABB16KSKF80,ABBH-22-LMGA,ABB12/18KLKF80P3,ABB16/22KSKF80,ABCIRH03JE,ABB18NPA,ABB 4 TKSK F80 P1 001,ABBMKM1050,ABCIRH03A..M6,ABBH - ** - ** - SUA - F80,ABCIRH00,ABCIRH07,ABCIRH05,ABCIRH04,ABB16KLKF80,ABCIRH03,MS 3057A,ABB16/14KLKF80P3,MS 3057C,ABB24NP,ABB-18-#GA-#M32,ABBH-10SL-LMGA,ABB16S/14KPKF80,SX - M6,ABB160100KPKF86,ABCIRH03FT,ABB-14S-#GA-#M32,ABB16/20KPKF80,ABCIRH03FM,ABCIRH03HC,ABCIRH03HD,ABCIRH03APC#,ABCIRHP08FT,ABBH - ** - ** - FT - F80,ABCIRH03GS,ABCIRHP08FM,ABCIRH03GM,ABB ET 16,ABBE - ** - X-PCA,ABB100/60KPKF80,ABB ** NP,ABB ET 12,ABCIRHPSE06T,ABBH-14S-LMGA,ABBH - ** - ** - FT F80,ABCIRHP06EV,ABCIRHP,ABB-40-#GA-#M32,ABB16/20KLKF80P3,ABB8/14KSKF80,ABCIRH03FC,ABB36NP,ABB4KPKF80,ABCIRH03EV,ABB - ** - KFP F80,ABB16S/20KPKF80,ABCIRH09RC,ABCIR-24-SX,ABB16/12KPKF80,ABB16S/14KPKF80,ABBH-20-LMGA,ABCIRHP06GGL.......,ABB12KSKF80,ABB600090,ABMRP31038,ABCIRHP06GGS........,M310,ABB16S/18KPKF80,ABB500/160KPKF8,ABCIRH00A/ABCIRH00A...M6,ABCIRH,ABCI03A,ABCIRH04T,ABBH - ** - ** - SUA*S,ABCIRH03F,ABB-10SL-#GA-#M32,ABCIRH03G,ABCIRH03D,CMSP3057A14S,ABB 4 CKPK F80 P1 002,ABCIRH06JE,ABB 4 CKPK F80 P1 001,ABB 4 CKSK F80 P1 001,ABB 4 CKSK F80 P1 002,X-PCAK&X-PCAJ,ABCIRH04EV,ABCIRH03M,ABBH - ** - ** - CCA - F80,ABCIRH03H,ABBH-####-LMGA-#,ABB40NP,ABCIR-22-SX,ABB160KSKF80,ABCIRH03T,ABCIRH03R,ABB16S/12KSKF80,ABB16/14KPKF80,ABB8/10KPKF80,ABB500/250KSKF8,ABBH - ** - ** - LMGA - F80,ABB12/16KSKF80,ABB14SNP,ABCIRH00XLC,ABCIRH00XLA,CMSP3057C10SL,ABB12/20KLKF80P3,ABB500/350KPKF8,ABB600061,FT8/WA27F,ABB16SKPKF80,ABCIRH06HC,CMSP3057A16S,ABCIRH06HD,ABCIRHSE06F2821PCNF80****,ABCIRH01M,ABCIRH05EV,ABB100/60KSKF80,ABB16/22KLKF80P3,ABCIRH01T,ABB600052,ABCIRH01R,ABCIRH06GM,ABB16/18KSKF80,ABB600057,ABCIRH06GS,ABB600058,ABCIRH00D,ABCIRH00A,ABB-36-#GA-#M32,ABCIRH12A,ABBIT16,ABCIRH00G,ABCIRH00H,ABCIRH00F,ABB20NP,ABCIRH00T,ABB4CKPKF80P1001,ABCIRH00R,ABB4CKPKF80P1002,ABB1210KSKF80,ABBIT12,ABCIRH00M,ABB-12-SGP,ABCIRH03APC#...M6,ABCIRH09JE,ABB16/18KLKF80P3,ABB24NPA,ABB4/10KPKF80,ABBTH619,ABB12/16KLKF80,ABCIRH06EV,ABB16/22KPKF80,ABB601200,ABB8KSKF80,ABB4TKSKF80P1001,ABB16KLKF80P3,ABB16S/20KSKF80,ABB12/20KPKF80,NPA,ABCIR-32-SX,ABBTH608,ABCIRHP08F,ABEB12/20KLKF80P3,ABBH - ** - ** - OCN - F80,ABCIRH08FT,ABB36NPA,ABCIRH08FM,ABBMBS020010,ABCIRH08FC,ABCIRH09HD,ABCIRH12A...M6,ABB14SNPA,ABCIRH09HC,ABB16SKLKF80P3,ABB20NPA,ABCIR-14S-SX,ABCIRH01XLC,CMSP3057C14S,ABB32NP,ABB8/12KPKF80,ABB12/40KSKF80,ABB4TKPKF80P1001,ABCIRH01XLA,ABB - ** - CRA,ABB16/12KLKF80P3,ABB4KSKF80,ABCIRHP06D,ABB-24-#GA-#M32,ABBH-24-LMGA,ABCIRHP06H,ABCIRHP06G,ABB12/14KSKF80,ABBH - ** - ** - SUA# - F80,ABB 4 TKPK F80 P1,ABCIRHP06R,ABBH - ** - ** - XLC - F80,ABB4CKSKF80P1002,ABCIRHP06T,ABB4CKSKF80P1001,ABB16S/14KSKF80,ABCIR-18-SX,ABB4/10KSKF80,ABB500/160KSKF80,ABB18NP,ABB12KPKF80,CMSP3057C16S,ABCIRH03APC#/AB,ABB8/40KSKF80,ABB500KSKF80,ABB12/10KLKF80P3,ABB16SNPA,ABB16S/14KSKF80,ABBM310,ABB500/350KSKF8,ABCIRHP06XLA,ABCIRHP06XLC,ABB4TKSKF80P1,CMSP3057A28,CMSP3057A32,ABB32NPA,ABCIRHP06RC,SBMSH55410,ABBH - ** - ** - FM - F80,CMSP3057A36,SBMSH55411,ABCIR,ABBH - ** - ** - HC - F80,ABB12/14KPKF80,ABB8/14KPKF80,ABCIRH09M,ABB 4 TKPK F80 P1 001,ABCIRH09H,ABBH-28-LMGA,ABB1614KPKF80,ABCIRH09T,ABCIRH09R,ABBD36,ABB12/40KLKF80P3,CMSP3057A10SL,ABB12/20KSKF80,GGS,ABB500/
TT Electronics Introduces 12V/24V High-Performance Dual Output Power Supply TAA 240-27C
TT Electronics today announced the launch of its new TAA 240-27C 12V/24V Dual Output Power Supply. This cutting-edge power supply delivers the highest output wattage on the market for a 12V/24V dual output, making it an ideal solution for a wide range of applications.
产品 发布时间 : 2024-08-23
TT Electronics 传感器和专业组件产品选型指南
目录- Company Introduction Optoelectronics Discrete Components Optoelectronics Standard Assemblies Optoelectronics hybrid Components Surface Mount and Chip Carrier OEM Pressure Sensors High-Reliability JAN Isolators/ COTS Fiber Optics VLEDs Steering Torque and Position Sensors Level Sensing Products Pressure Transducers and Transmitters Flush Mounting & Abrasive Media Submersible Transducers and Transmitters Flowmeters Temperature Instrumentation Calibration Services
型号- OP604ESA-B1,OP604ESA-B2,OP803TX,PB-AIVAC-0001-C-G,OP224,OPB930W51Z,OMH3019,3C91CTX,OPB700ALZ,P170SP1,OMH3131,OP223TX,OPB871P51TX,P270,OPB970N51,OPR5011,OMH304,2N2222AUBTXV,43LF,HCC242,SX-4431,OPB847TXV,OPB872N51TX,SX-4432,OP803SL,OPB872T55TX,HCC240,SX-4433,GPB-AIVAC-005-D-G,P160KNPD,OPI1264B,OPI1264A,OPI1264C,OPF372A,OMH307,OPB871P51TXV,OP235TX,OPB870N51TXV,2N2222AUATXV,A,OPB971N51,B,OPV332,C,D,SX-4300,E,PB-AIVAC-0001-B-G,4N49UTX,SX-4428,SX-4429,OPB870P11TX,OPB390L11Z,OPB811W55Z,5711,OPI150,OPB810W51Z,5713,OPB983T51Z,OP224TX,OPB870L11TXV,OPR5910T,OP535B,OPB848TX,P170SPD,PB-AIVAC-001-A-G,OPB815WZ,PSXX-2,OPB872L55TXV,SX-4413,OPF2412,SX-4414,PSXX-1,SX-4417,OPB871T51TXV,OP236TX,2N5794UTXV,OPB870N11,OPB872T11TXV,OPL550,OPI1268,OMH3150B,OPI1266,OPB350W062Z,SX-4487,PB-AIVAC-0001-A-G,OPB930W55Z,OPB848TXV,OPB885Z,OMH3040S,OPB870T11TXV,OPB704WZ,OPB871N55TXV,PB-AIVAC-005-E-G,OPR5011T,5611,5613,OMH3040B,OPB847TX,SX-4475,SX-4476,SX-4473,OP505A,SX-4474,SX-4479,OPB872T11TX,OPB100-SZ,PB-AIVAC-001-B-G,OP805SL,OMH3075S,OP132,OPB365T55,3C92CTX,OPB872T55TXV,OPB990T51Z,OPB701ALZ,OPB730F,OPB350,OPB810L51,OMH3075B,OPB872L55TX,OP804TX,OPB200,2N6989UTX,SX-440,OPB870P55TX,2N5794U,OPB871L51TXV,P170N2,OPB822SD,OVS5MGBCR4,OPB961N51,OMH3150,EN12-HS,OP604ESA-C2,OP604ESA-C3,PSXXM,PSXXL,EN16-H,PB-AIVAC-001-C-G,OP232,4N48UTX,OP233,EN16-V,OPF370A,5401,PB-AIVAC-002-A-G,OMH3020,5403,OP805TX,4N23(A)(U),OPB821TXV,OPB872P51TX,OPB980L55Z,OP804TXV,OP224S,OPB825,OPB704,OPI110C,OPB982P51Z,OP181,OPB870P55TXV,OPB832W55Z,OPB871T55TX,OPB711,OP603TX,OP265FAB,5311,2N2222AUB,2N2222AUA,OP604TXV,OPB870N11TX,3371,OPB990P51Z,7601,7603,OPI150TX,OPB870T51TXV,OP234W,OP603TXV,OPB804,OVSRRGBCC3TM,OPB829DZ,OPB961T51,OPB992T51Z,OVQ12S30W7,P120PK,OPB872N55TX,P170NP1,OP550A,OPB821TX,EN11-HSM,OPB720B-30VZ,OPR2100HST,OP602TX,OMH090S,OPB818,OPB829CZ,EN11-HSB,P140KH,OPB700TX,OMH090B,HCC242TXV,OPB821S5Z,OPV310Y,OPB745,6671,6673,5101,OPB620,5103,P160KN/KN2,HCT7000MTXV,OPB625,SX-4404,PS45G,OPB627,OPB890P51Z,OPB733TR,OH090U,GPB-AIVAC-002-D-G,OPB831W55Z,OPB983P51Z,OPB970N11,4N24(A)(U),OPB870N55TX,OPB871P55TXV,HCC240TXV,OPB910W55Z,OPL530,OPV314,OPB100Z,OPB872P55TXV,3N261TX,OPB870P51TX,EN11-VSM,OPB755TZ,OPI120TXV,OPB871L51TX,OPR5925,OPB390P11Z,OPB870P11TXV,2N5794UTX,OPB610,OP604TX,OP803TXV,OPB730,4N22(A)(U),2N4854UTX,OPI123,2N6989UTXV,OPI125,OPR5915,OPI126,OPI127,OPR5910,OPR5911,OPV314Y,OPR5913,OPI120,OP906,OPB820S10,4N24UTXV,3N262TX,2N6987URXV,OPB870T55TX,OPB608B,OPB980T55Z,OPB608A,OPB608V,OPB870L11TX,OPB871T51TX,2N2222AUATX,2N4854UTXV,PB-AIVAC-0004-E-G,6121,6124,6126,OPB911W55Z,OPB706A,OPB872N55TXV,PB-AIVAC-003-D-G,4N47UTXV,OPB870L51TXV,OPB870N11TXV,OPB718Z,OPB872T51TXV,OP223TXV,4N22U,OP593A,OPB830W55Z,OPB815L,OPB871L55TXV,6373,22,23,P090S,7221,25,7223,4N22UTX,3N263TX,P090L,OPB870N51TX,6371,OVSARGB4R8,OPB390T51Z,OPB680-20,OPB872P51TXV,4N47U,7216,6127,OPL800TX,OP166A,2N2222AUBTX,PB-AIVAC-0004-D-G,OPB903W55Z,6143,OPB871N51TXV,OP913WSL,OPB840W11Z,PB-AIVAC-003-E-G,OPB716Z,OPB872P55TX,OPB732WZ,4N48U,OP235TXV,OP240B,OP240A,4N24U,6138,3C92CTXV,6153,44,6273,9301,7483,45,OP245A,7486,3C91CTXV,9303,GPB-AIVAC-010-D-G,OP232W,P09XS,OPB870T11TX,OPB891T51Z,7481,P09XN,4N23UTXV,OPB870L55TX,4N49U,OVS5MBBCR4,OP245B,OPR5500,OPB912W55Z,OPI120TX,PB-AIVAC-0004-C-G,6163,OPB460N11,OPB840L11,2N2907AUBTX,2N5796UTX,P170NPD,4N48UTXV,OP805TXV,OP233W,OPB880P51Z,OPR2100,OMH3019S,OPR2101,62,OPB830W11Z,2N4854U,64,66,67,68,7386,OPB390T55Z,OP133W,OPB350W125Z,OP266W,OPB828D,OPR2100HS,OMH3019B,6173,7383,OPB870N55TXV,OPB816Z,HCT802TXV,OPB871L55TX,OPB870T55TXV,72,OPF482,OPB872L51TXV,6186,7274,78,7276,OPB380P55Z,OPB933W51Z,PB-AIVAC-0004-B-G,OPB840W55Z,OP602TXV,OPR2100T,OPB871T55TXV,OPB871N51TX,82,83,84,OPB100-EZ,OPB870T51TX,7286,OHB3040,7288,SX-4289,4N47UTX,4N24UTX,3N243TX,OPB913W55Z,OPI150TXV,2N5796U,OP224ESA-B2,OPB973N51,OP224ESA-B1,OP980,7284,OP224ESA-B3,OMH3020S,EN12-VS,OPB991L51Z,4N49UTXV,PB-AIVAC-005-C-G,OPB720B-12Z,93,8146,OHB3040B,OPB871P55TX,OMH3020B,PB-AIVAC-0004-A-G,8148,OPB880T51Z,PB-AIVAC-001-D-G,4N22UTXV,OP224ESA-C1,OP802WSL,OPB872T51TX,OP224ESA-C3,OP224ESA-C2,PB-AIVAC-010-A-G,OPB800W51Z,2N2907AUBTXV,OHB3040S,PB-AIVAC-002-B-G,OPB990T55Z,OPB710F,OP800A,OPB940W51Z,HCT802TX,8156,OPR5200,8154,OPL800TXV,SX-4388,P110KV/KVI,OP506B,OP506A,HCC240TX,3N244TX,8152,4N48(A)(U),P08XS,OPB943W51Z,8151,OVSRRGBCC3,P08XN,OPB700TXV,2N2907AUATXV,2N5796UTXV,OP291A,GPB-AIVAC-010-E-G,OPB800L55,PB-AIVAC-005-B-G,OPB871N51,SX-4491,OPB840W51Z,OPB811L55,4N49(A)(U),PS60G,P170S,OPR2800V,OPB870L51TX,PB-AIVAC-001-E-G,OPR2800T,2N2907AUATX,P170N,OP950,PS100-2,OMH3150S,PS100-1,OP604S,PB-AIVAC-003-A-G,P170S2,P09X5N,PB-AIVAC-002-C-G,OPB901W55Z,OP580DA,OPB842W51Z,2N6987UTX,OPB990L51Z,PB-AIVAC-0001-E-G,3N245TX,OPB857Z,P160KNP,OPB822S,2N2907AUA,PB-AIVAC-005-A-G,2N6987U,OP236TXV,OPB870P51TXV,2N2907AUB,OP140A,P230,OPB870L55TXV,OPB941W51Z,4N47(A)(U),OVS5MRBCR4,P261P,PB-AIVAC-010-C-G,P140KH1,PB-AIVAC-003-B-G,OP298A,OPI125TXV,OPB350L062,OPB872N51TXV,OPB871N55TX,OP225TXV,OPF522,P260T,OPB872L51TX,PB-AIVAC-0001-D-G,OPB900W55Z,P260S,P260P,89/90,OPB350W250Z
TT Electronics(TT)专业音频应用的面板电位器/编码器/滑动电位器选型指南
目录- Panel Potentiometers and Encoders Product Introduction Panel Potentiometers Encoders Slide Potentiometers Panel Potentiometers/encoders/Slide Potentiometers Applications
型号- P140KV,EN12-VS,P170SP1,P272,P271,P170SNPD,P270,EN12-VN,P160 SERIES,P265S,P160KNPD,P265P,P120K,P09X SERIES,P260,P09X,EN09H1020ASF012,P110KV1,P090S,P165,P090L,P110KH,P120PK,EN09H1020ANFW012,P170NP1,EN11-HSM,P170SPD,LM1001,PSXX-2,P110KV,P278,P170S,PS60G,PSXX-1,P140KV1,P170N,P166V,EN11-VNM,PS100-2,EN11-HSB,PS100-1,P166P,P140KH,P170S2,P162,P161,P166H,P270 SERIES,P164,EN11-VNB,P09X5N,P163,P160,P232 SERIES,P231 SERIES,EN11-HNB,P090S SERIES,P09XS,EN05VS,P230 SERIES,EN05VT,PSL2,P160KNP,PS45G,P09XN,P230,PSS1,PSS2,P261S,EN11,EN12,P261P,EN16,P160KN,P140KH1,P261D,P260 SERIES,P090 SERIES,EN09V1020ASKS115,P260T,P260S,P260P,EN11-VSM,P170N2,P090,P260D,EN12-HN,EN05HS,EN11-VSB,EN09B1020ANKW200,EN12-HS,P232,PSXXM,P231,P233,PSXXL,EN16-H,P110KH1,P090L SERIES,EN16-V,EN11-HNM
TT Electronics (TT)电阻器选型指南
目录- Technology and Product Selector Guides Current Sense Resistors High Value/Voltage Resistors Surge/Pulse Resistors High Power Resistors Precision/Fusible Resistors High Reliability Resistors Temperature Sense & Thermal Management Resistors Zero-Ohm Jumper & General Purpose Resistors Colour Code for Band-marked Resistors
型号- BCN168SB,WH SERIES,T 2010,WP25S,WMO7S,BCN168RB,0612X,BCN,WP5SZI,WHS-UL,WIN SERIES,RCP,SQM5,SQM3,SQM2,EBW5216,N 0815,LRF SERIES,SQM7,4507,LRCS,4500,WMO5S,4501,4502,4503,4504,VRW37,4505,4506,RHVD08,RC70P,T0603,WRM SERIES,HDSC,CR,LVC SERIES,VRW25,DIV23 SERIES,ULW SERIES,RHVD15,LRMAPXXXX SERIES,4530,GHVC SERIES,ULR3N,4531,WDBR-UL,TJC,RHVD10,RC65,WMO3S,BPR50,WH10,ULR,EMC,LCS SERIES,ULW,WP-SZI SERIES,WHPC,PCF,WMO2S,RC55,4532,4533,4534,4535,4536,4537,PCR,HPDC SERIES,OAR,SQP SERIES,WPYP10,ULR1S,BCN10,WSHR,ULR25,WRM,1900 SERIES,W20,WMO1S,W22,W21,T0805,W24,W23,SQP7,SQP5,ULR2N,BPR30,SQP2,SQP3,SOT143 SERIES,RC70,LR SERIES,WSM,HVC,W31,W30,HVD,WHS2,WHS3,WHS5,W20 SERIES,WH25,WHS7,WP3SZI,BPC3,RC65P,HVP,WH50,LHVC,HR,PFC,WMO-S SERIES,VRW,MFP1,MFP2,BPR10,WA84F,EBW SERIES,MF-S,WP-SZI,BPC7,BPC5,HVP SERIES,MFC SERIES,WSM2,WSMHP,WSM3,GHVC2512,MF1/2S,WCM SERIES,BPC10,LOB-5,ULR15S,LOB-3,MFR5,LRF3W SERIES,MFR3,MFR4,HVD08,RHVD10A,WH100,RC SERIES,VRW SERIES,SOT,RC55P,WSMHP25,HVD15,LR,WSMHP20,HVD12,1020X,DIV23,SPP,WMHP35,DSC,0508X,WRM-HP SERIES,WP-S SERIES,MF2S,LOB SERIES,MFR SERIES,HVD20,WHS10,WH200,WMHP50,SQM,PFC-HT,LRZ SERIES,SQP,BPC,LRMA,WSMHP SERIES,OARS-XP SERIES,LCS,MF1S,EMC SERIES,PR SERIES,BPR,WMHP20,N 0612,MFC,HVD30,RHVD15A,PCFH,T43,OARS-XP,T44,MFP,MFR,PCFP,T48,WCR SERIES,WA84F SERIES,WSMHP35,4530 SERIES,WH300,T0402,APC,HPWC,MF3S,VRW68,PR,WPYP SERIES,LRCS SERIES,OAR-5,OAR-1,OAR-3,1908,RC,PCR SERIES,RHVD SERIES,PCF SERIES,WHS5UL,1906,1905,1900,N 1225,BCN SERIES,PWC SERIES,1600 SERIES,LRF3W,ASC,WHPC SERIES,MFP SERIES,HTCR,PWP08,RCP SERIES,CAR SERIES,APC SERIES,WPYP8,P4026,PR5,PR4,TJC SERIES,WMHP,WP-S,SQM SERIES,SPP SERIES,PWP04,PWP06,OARS1,GHVC1206,T1206,OARS SERIES,HVP08,HVP06,TFHP SERIES,HVP04,WHS-UL SERIES,WRM-HV SERIES,PTS SERIES,HVD SERIES,M 2512,WSM SERIES,P 3920,WMO1/2S,WHS SERIES,SC3 SERIES,HVP15,ULR SERIES,HVP10,SPP-1,T 2512,LHVC SERIES,SPP-3,PTS,SPP-2,ULR2,ULR1,WH,WP2S,BPR SERIES,EMC2,WDBR1/2,ULR5,TFHP,HVP20,ULR3,M 1206,WL4 SERIES,BPR7,0207HP,BPR5,1602,BPR3,1601,SP20F SERIES,1600,WP1S,WPRT,WRM-HV,GCR,1609,1608,1607,1606,1605,SP20F,1604,1603,WRM-HP,WCM,WMHP SERIES,WCR,BPC SERIES,PFC-D,BCN164A,WP4S,WDBR5,WDBR SERIE,WDBR7,WDBR2,CAR7,PWC,WDBR1,CAR6,LOB,CAR5,WDBR3,PWP,P 2512,WP3S,BCN164AB,WPRT10,WPRT15,M 0805,P2817,OARSXP,RHVD,P 2726,WMO-S,WPRT20,EBW,3810,3811,WP2SZI,3812,CR SERIES,S0M10,DSC SERIES,WMHP100,GHVC,4800 SERIES,WPRT30,WP5S,HTCR SERIES,LRF,WH5,LRMA SERIES,P 5930,4804,4805,WPRT40,LRZ,GHVC2010,GCR SERIES,WPYP,PWP10,4800,PWP15,4802,WHS,HPDC,EBW8518,MF-S SERIES,OAR SERIES,PWP SERIES,4500 SERIES,ULW5,ULW4,ULW3,ASC SERIES,WPRT50,CAR,ULW2,OARS,PWP20,WHS3UL,W30 SERIES,4812,WIN,SQP20,SQM10A,LRMAPXXXX,3810 SERIES,WPRT SERIES,SOT143,HR SERIES,HVC SERIES,SQP15,SC3,SQP10,WDBR,WHS2UL,LVC,PFC-D SERIES,4832,WL4
【成功案例】TT Electronics非接触式流体传感器成功应用于气泡检测
TT Electronics的非接触式流体传感器具有免受环境照明影响的优点,具有电路板安装和电线安装两种方式,兼容多种管径尺寸,能够实现对液体的非接触式测量。本文应用于医疗器械体外诊断行业,通过选择合适尺寸的TT Electronics的非接触式流体传感器OPB350W125Z,设计恒流源驱动等硬件电路,实现稳定的气泡检测功能。
应用方案 发布时间 : 2019-03-26
TT Electronics光纤选型表
提供TT Electronics光纤发射器,光纤接收器,光纤探测器选型,波长涵盖840nm/850nm/860nm,温度高达125℃。
产品型号
|
品类
|
Wavelength(nm)
|
Operating Temperature(℃)
|
Continuous Forward Current(mA)
|
Maximum Reverse Voltage(V)
|
Forward Voltage(V)
|
OPF1414
|
High Speed Fiber Optic Transmitter
|
840nm
|
-40℃~85℃
|
100mA
|
1.8V
|
2.09V
|
选型表 - TT Electronics 立即选型
【选型】TT Electronics(TT)ABCIRP模块化卡口锁连接器选型指南
目录- Company Profile and Modular Bayonet Lock Connector Introduction Technical Information and Part Number Explanation Insulators Seal Grommet Crimp Contacts Receptacle/Plug Connector Locking Backnut Adapter/Extender Cable Clamp and Bushing Gasket and Nut Plates Receptacle Mounting Detail Metal Protective Caps and Braid Strap Connector Supplementary Tooling Connector Safety Information
型号- ABCIRPSE22FS,P80 16 GET,ABCIRP12/14KPKP3,ABB-24-#GA-#M32,ABCIRP40-A60GSS,CMSP3057C**M32,ABBP8/16KSKP80,ABCIRP16/14KPKP3,ABCIRP40-A60GSL,ABCIRP16/22KLKP3,ABCIRP24-10PFS,ABCIRP28-21GSL,P8028GAT,ABBE**XPCAK,ABBP100KPKP80,ABCIRP12/40KLKP3,ABCIRP12/10KLKP3,ABCIRP18-19S,ABCIRP20-15GPL,ABCIRPPSE06T,ABCIRP03T,ABBMBS035015,ABCIRP40-A60S,ABCIRP24-10S,FT8,ABBP**/**KPKP80,ABCIRP20-15GPS,ABCIRP22-14PFS,ABCIRP32-A13GSL,ABBE**XPCA,ABCIRP22XR,ABCIRP36-A22S,ABCIRP32-A13S,ABCIRP12/18KLKP3,68-016-01,ABCIRP32-A13GSS,P80 8 ETA,ABCIRP18-19GPL,ABBP100KSKP80,ABCIRP22-14GPS,ABCIRP22-14GPL,ABCIRP32-A13GPS,ABCIRP36-A22GPS,ABCIRP12KLKP3,ABCIRP28XR,ABCIRP20-15S,ABCIRP24XR,SB ** RPGS,ABCIRP12/20KPKP3,ABCIRP36IC,ABCIRP36-A22GPL,P80 16 ITL,ABCIRP18-19PFS,ABB608109,ABCIRP20IC,ABCIRP28-21PFS,ABCIRP40-A35PFS,ABCIRP40-A60PFS,ABBD51,ABBMBS050015,ABCIRP**/**KLKP3,ABCIRP16/20KPKP3,ABB12KFPF80,ABBPL0550A1130,ABBP8/12KPKP80,ABCIRP16/18KLKP3,P80 16 ETA,ABCIRP32-A13GPL,ABB600061,6M3800644,ABCIRP28-21GSS,ABCIRP28IC,ABCIRP,6M3800645,ABBP100/60KPKP80,ABB-20-#GA-#M32,ABCIRP24IC,6M3800646,ABCIRP09T,ABBMKM1050,ABB600052,67-012-01,SBMSH554,ABB600057,ABCIRP24-10GPL,ABB600058,AB-CIRP03LBN2214GPSPFS****VON,WA27F,ABCIRPSE24FS,ABCIRP22-14S,ABBP100/60KSKP80,ABCIRP16/22KPKP3,P80 12 ITL,ABCIRPSE20FS,ABCIRP40-A35GSS,6M3800730,6M3800731,ABCIRPSE28FS,ABCIRP24-10GPS,ABCIRP18IC,ABB-18-#GA-#M32,ABMRP0925,ABB8KFPF80,ABBTH592,ABCIRP32XR,P80 12 ETA,ABCIRP18-19GSL,ABCIRP40IC,ABCIRP18-19GSS,P8018GAT,ABCIRP40-A35GSL,ABCIRP12/40KPKP3,ABBP**/**KSKP80,ABB16KFP,ABCIRP20-15PFS,ABCIRPSE36FS,ABCIRP16/18KPKP3,ABCIRPSE32FS,ABCIRP40-A60GPS,ABCIRP12/10KPKP3,ABCIRP36-A22PFS,ABCIRP40-A60GPL,SB ** FPGS,ABCIRP36XR,ABCIRP28-21S,ABCIRP20XR,ABCIRP32IC,ABBMBS025015,68-012-01,ABCIRP12/18KPKP3,SBMSH554**,ABB601200,ABCIRP8/40KPKP3,ABBP8KSKP80,SB ** FPG,ABCIRP20-15GSL,ABCIRPSE06T,ABCIRP18-19GPS,ABCIRP28-21GPS,ABCIRPSE40FS,ABCIRP20-15GSS,CMSP3057A,SB ** RPG,ABCIRP12/20KLKP3,CMSP3057C,ABCIRP28-21GPL,ABBP8KPKP80,CMSP3057A**M32,ABCIRP24-10GSL,ABCIRP12KPKP3,ABCIRP22IC,ABCIRP16/20KLKP3,ABBMBS020010,P80 12 GET,HT250-3,ABCIRP12/16KPKP3,ABCIRP16KPKP3,TH592,ABBP8/16KPKP80,ABCIRP22-14GSS,D51,ABCIRP36-A22GSS,ABBFT8,ABCIRP40-A35GPS,ABCIRP22-14GSL,ABCIRP**/**KPKP3,ABCIRP24-10GSS,ABWA27F,ABCIRP36-A22GSL,ABCIRP18XR,ABCIRPSE18FS,ABB**EPCA,ABB**EPCAK M32,ABCIRP40-A35S,ABBP8/40KSKP80,ABBP8/12KSKP80,ABCIRP32-A13PFS,ABB-32-#GA-#M32,ABCIRP40XR,ABCIRP12/14KLKP3,ABCIRP40-A35GPL,67-016-01,ABB600090
【选型】TT Electronics(TT) 汽车级电阻选型指南
目录- AEC-Q200 认证的电阻
型号- LRMAP2512,LRMAP3920,PCF0402,WSMHP25,PCF0603,LR,OARS1,WSMHP20,WCR1210…A,CHP,LHVC,WCR2512¡�A,PCF1210,WCR…A,WPRT40,LRZ,CHP2,CHP1,CHP1/2,ULR,LR(F)2512,CHP1/8,PCF1206,LRZ2010,LHVC2010,LR1206,LR2010,PCF-H,WCR0402…A,LRZ1206,PCF1210H,PCF2010,WCR0805…A,LRMAM1206,BCN,WHPC,PCF,PCF1206H,WCR1210¡�A,WPRT50,BCN164AB,OARS,LRMA,WPRT10,WPRT15,WCR0603…A,OARSXP,WCR2512…A,WCR0805¡�A,WSMHP,WCR2010¡�A,LRMAT2512,ULR25,HVC2512,ULR2,LRF3W,PCF2010H,ULR1,LR(F)2010,PCF0805H,LR(F)1206,WPRT20,PCF0603H,LRMAN0815,ULR3,WCR1206…A,LR2512,SC3,WSMHP35,LRZ2512,PCF2512,WHPC2010,WPRT,HVC,OARS3,PCF0805,WCR1206¡�A,WCR0603¡�A,WHPC1206,WCR0402¡�A,WPRT30,WCR2010…A
TT Electronics Opens New Manufacturing Facility in Mexico
TT Electronics proudly announces the grand opening of its newest manufacturing facility located in Mexicali, Mexico. This strategic expansion marks a significant milestone in the company‘s continued growth and commitment to serving customers worldwide.
原厂动态 发布时间 : 2024-02-23
电子商城
品牌:TT Electronics
品类:High Power MELF Resistors
价格:¥0.5375
现货: 9,000
品牌:TT Electronics
品类:High Power MELF Resistors
价格:¥0.5375
现货: 9,000
品牌:TT Electronics
品类:High Power MELF Resistors
价格:¥0.3305
现货: 9,000
品牌:TT Electronics
品类:High Power MELF Resistors
价格:¥0.3305
现货: 9,000
品牌:TT Electronics
品类:High Power MELF Resistors
价格:¥0.5375
现货: 9,000
品牌:TT Electronics
品类:High Power MELF Resistors
价格:¥0.3305
现货: 9,000
品牌:TT Electronics
品类:High Power MELF Resistors
价格:¥0.5375
现货: 9,000
品牌:TT Electronics
品类:High Power MELF Resistors
价格:¥0.5375
现货: 9,000
品牌:TT Electronics
品类:High Power MELF Resistors
价格:¥0.5375
现货: 9,000
品牌:TT Electronics
品类:High Power MELF Resistors
价格:¥0.5375
现货: 9,000
现货市场
品牌:TT Electronics
品类:Low Resistance Metal Alloy Resistors
价格:¥0.7268
现货:160,000
品牌:TT Electronics
品类:Low Resistance Metal Alloy Resistors
价格:¥0.7268
现货:107,900
品牌:TT Electronics
品类:Wirewound Power Radial Terminal Resistors
价格:¥29.9250
现货:22,389
品牌:TT Electronics
品类:Wirewound Power Radial Terminal Resistors
价格:¥7.1649
现货:814
品牌:TT Electronics
品类:Wirewound Power Radial Terminal Resistors
价格:¥16.0569
现货:42
品牌:TT Electronics
品类:Wirewound Power Radial Terminal Resistors
价格:¥17.9550
现货:7
服务
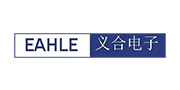
可贴片PCB尺寸50*50mm-580*610mm;PCB厚度0.3-8mm;贴装精度CHIP元件+0.03,BGA Pitch 大于0.25mm;元件尺寸0201-74*74BGA;元件高度:30mm。
最小起订量: 1 提交需求>
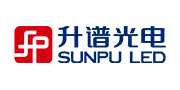
可定制LAMP LED、 CHIP LED、 PLCC LED、 汽车用车规级LED、COB LED的尺寸/电压/电流等参数,电压1.5-37V,电流5-150mA,波长470-940nm。
最小起订量: 30000 提交需求>
登录 | 立即注册
提交评论