LTE Physical Layer Overview




There are two types of frame structure in the LTE standard, Type 1 and Type 2. Type 1 uses Frequency Division Duplexing (uplink and downlink separated by frequency), and TDD uses Time Division Duplexing (uplink and downlink separated in time).
This overview is not an exhaustive description of the physical layer but is intended to provide you with a useful background when you configure the 89600 VSA LTE demodulator to make measurements.
Terminology
First, an introduction to some of the terms used in describing an LTE Frame. There are six-time units: frame, half-frame, subframe, slot, symbol, and the basic time unit (Ts), as shown in the following table.
Below is an illustration of an FDD frame.
A resource block (RB) is the smallest unit of resources that can be allocated to a user. The resource block is 180 kHz wide in frequency and 1 slot long in time. In frequency, resource blocks are either 12 x 15 kHz subcarriers or 24 x 7.5 kHz subcarriers wide The number of subcarriers used per resource block for most channels and signals is 12 subcarriers.
The 89600 VSA LTE demodulator currently only supports resource blocks that are 12 subcarriers wide.
Frequency units can be expressed in a number of subcarriers or resource blocks. For instance, a 5MHz downlink signal could be described as 25 resource blocks wide or 301 subcarriers wide (DC subcarrier is not included in a resource block).
The underlying data carrier for an LTE frame is the resource element (RE). The resource element, which is 1 subcarrier x 1 symbol, is the smallest discrete part of the frame and contains a single complex value representing data from a physical channel or signal.
The bandwidths defined by the standard are 1.4, 3, 5, 10, 15, and 20 MHz. The table below shows how many subcarriers and resource blocks there are in each bandwidth for uplink and downlink.
For downlink signals, the DC subcarrier is not transmitted but is counted in the number of subcarriers. For the uplink, the DC subcarrier does not exist because the entire spectrum is shifted down in frequency by half the subcarrier spacing and is symmetric about DC.
FDD frame type 1
In FDD mode, uplink and downlink frames are both 10ms long and are separated either in frequency or in time.
For full-duplex FDD, uplink and downlink frames are separated by frequency and are transmitted continuously and synchronously.
For half-duplex FDD, the only difference is that a UE cannot receive while transmitting.
The base station can specify a time offset (in PDCCH) to be applied to the uplink frame relative to the downlink frame.
TDD frame type 2
In TDD mode, the uplink and downlink subframes are transmitted on the same frequency and are multiplexed in the time domain. The locations of the uplink, downlink, and special subframes are determined by the uplink-downlink configuration. There are seven possible configurations given in the standard. The following is an illustration of a TDD frame with an uplink-downlink configuration set to 2 and a special subframe configuration set to 6.
Special subframes
Special subframes are used for switching from downlink to uplink and contain three sections: DwPTS, GP, and UpPTS.
DwPTS is the Downlink Pilot Time Slot. DwPTS contains P-SS. PDSCH can also be transmitted during DwPTS when DwPTS is configured to be longer than a slot.
UpPTS is the Uplink Pilot Time Slot. UpPTS can contain PRACH and SRS, but cannot contain or PUCCH or PUSCH.
GP is a guard period between DwPTS and UpPTS. PRACH format 4 begins in the guard period. Otherwise, nothing else is transmitted during the guard period.
The lengths of these three sections are determined by the special subframe configuration index (specified by the Dw/Gp/Up Len parameter). There are 9 possible configurations.
UL/DL configuration
The uplink-downlink configuration (specified by UL/DL Config) of a TDD frame determines the locations of uplink, downlink, and special subframes. There are 7 possible uplink-downlink configurations.
Subframes 0 and 5 and DwPTS in TDD frames are always allocated to downlink transmissions.
UpPTS and the subframe after a special subframe are always allocated to uplink transmissions.
Subframe 1 is always configured to be a special subframe. Subframe 6 can also be configured to be a special subframe.
Uplink
Uplink user transmissions consist of uplink user data (PUSCH), random-access requests (PRACH), user control channels (PUCCH), and sounding reference signals (SRS).
FDD and TDD uplink transmissions have the same physical channels and signals. The only difference is that TDD frames include a special subframe, part of which can be used for SRS and PRACH uplink transmissions.
The following illustration shows part of an LTE uplink frame and contains an allocation for each type of uplink channel. The illustration is applicable to both TDD and FDD.
User 1 has a PUSCH allocation of [RB 20, slots 4-5], and User 2 has a PUCCH allocation of [subframe 2, PUCCH index 0]. User 3 has been given an SRS allocation of subcarrier 94 to 135 in subframe 2, and User 4 is transmitting in a PRACH allocation.
A user cannot transmit both PUCCH and PUSCH data in the same slot.
TDD
In TDD mode, uplink and downlink transmissions occupy the same frequency spectrum but are separated in time. Uplink users transmit during subframes configured for uplink. In addition to uplink subframes, UE's can transmit random-access requests (PRACH) and SRS during the UpPTS section of the special subframe.
FDD
The uplink FDD frame is the same length as the downlink frame and contains only uplink user transmissions.
Modulation
For uplink data signals (PUSCH), the LTE standard uses Single Carrier - Frequency Division Multiple Access (SC-FDMA) modulation, which has a lower peak-to-average power ratio, meaning lower cost amplifiers and less power usage.
In SC-FDMA, the user data is modulated onto a single carrier modulation format (QPSK, 16QAM, or 64QAM), and the time domain symbols are transformed to the frequency domain by an FFT. Then the frequency domain points are mapped onto the subcarriers assigned to the user in the OFDM symbol. Finally, an IFFT is performed on the entire OFDM symbol, and the resulting time data is transmitted. The following image illustrates this process.
The value M in the M-point FFT in the illustration above is the width (in subcarriers) of the uplink allocation assigned to the user. Typically a UE is not allocated the entire spectrum, therefore M is less than or equal to N.
For symbols that contain demodulation reference signals, PRACH, PUCCH, or SRS, the uplink transmitter places the modulation symbols directly onto the OFDM subcarriers, performs the IFFT, and transmits the data in a manner similar to downlink OFDMA.
Synchronization
Uplink signals do not have a dedicated sync signal. In a real-world environment, the uplink signals would be synchronized using the downlink signal. However, to allow analysis of uplink separate from downlink when using the 89600 VSA LTE demodulator, uplink frames can be synchronized using PUCCH DM-RS, PUSCH DM-RS, PRACH, or SRS.
Reference signals
There are two types of uplink reference signals, the Demodulation Reference Signal (DMRS) and the Sounding Reference Signal (SRS). Reference signals are used for channel estimation or equalization.
DMRS
The Demodulation Reference Signal (DMRS) is used by the base station to equalize and demodulate the UE's transmissions.
The PUSCH demodulation reference signal is a Zadoff-Chu sequence, which results in constellation points on a circle centered about the origin.
The PUCCH demodulation reference signal, however, is a reference sequence transmitted on a rotated QPSK constellation. The amount of rotation is determined by cyclic shift (a) as defined in the standard.
Each uplink user transmits a Demodulation Reference Signal during certain symbols in each resource block allocated to the user. DMRS is transmitted on all subcarriers allocated to the user during the symbols listed in Table 5.5.2.2.2-1 in 3GPP TS 36.211.
SRS
The sounding reference signal (SRS) is transmitted separately from PUCCH and PUSCH. SRS can be transmitted on any number of subcarriers in the last symbol in an uplink subframe whether or not the subcarriers are assigned to another channel. The exception is that PRACH transmissions and PUCCH Format 1 and 2/2a/2b transmissions take precedence over SRS transmissions.
SRS is transmitted by a UE to give the base station an idea of the channel characteristics for that UE. The base station can use the information to assign good uplink allocations for the UE to transmit on.
Physical channelsPUCCH
There is only one control channel transmitted by uplink users—the Primary Uplink Control Channel (PUCCH)—which contains information including channel quality info, acknowledgments, and scheduling requests.
PUCCH is assigned by subframe instead of by slot. The location of PUCCH in the first slot alternates in the pattern:
Resource block 0, N-1, 1, N-2, 2, N-3, ...
where N is the frequency width of the frame in units of RB.
The location of the PUCCH resource block in the second slot is the one that mirrors the location of the resource block in the first slot. For example, in a 5 MHz uplink LTE signal, a PUCCH allocation of (subframe 1, PUCCH index 0) means that PUCCH is transmitted on (Slot 2, RB0) and (Slot 3, RB24).
The PUCCH index (set by the First RB parameter) determines which resource block in the first and second slots of the subframe is used for PUCCH.
PUCCH's modulation format is determined by PUCCH type and can be QPSK, BPSK, On/off keying, or a combination of QPSK and BPSK. See the PUCCH Frame Summary topic for a table of PUCCH types and their corresponding modulation formats.
PUSCH
The Primary Uplink Shared Channel (PUSCH) is used by uplink users to transmit data to the base station. All subcarriers not allocated for PRACH, PUCCH, or SRS are available for assignment to users. PUSCH data is modulated using SC-FDMA.
PUSCH data is transmitted using QPSK, 16QAM, or 64QAM as the single carrier modulation type before spreading (FFT, subcarrier mapping, then IFFT).
PRACH
The Physical Random Access Channel (PRACH) is used by an uplink user to initiate contact with a base station. The base station broadcasts some basic cell information, including where random-access requests can be transmitted. A UE then makes a PRACH transmission asking for PUSCH allocations, and the base station uses the downlink control channel (PDCCH) to reply where the UE can transmit PUSCH.
PRACH consists of a cyclic prefix followed by the PRACH sequence. There are five PRACH preamble formats with various CP lengths and sequence lengths. Format 4 is short enough to be transmitted during the GP and UpPTS sections of the special subframe and is only applicable to the TDD frame type. PRACH preamble formats are listed in Table 5.7.1-1 in 3GPP Technical Specification 36.211.
In the frequency domain, PRACH spans 6 resource blocks of the spectrum. PRACH formats 0, 1, 2, and 3 have a tighter subcarrier spacing of 1.25 kHz. This means that the OFDM symbol duration is 800 ms. PRACH format 4 has a subcarrier spacing of 7.5kHz and a symbol duration of ~133ms.
The frequency location of PRACH is determined by upper-layer parameters.
Downlink
The downlink frame contains the information being sent to the users that are currently connected to the base station. The frame also contains physical signals for synchronization (P-SS, S-SS), channel compensation (C-RS), and control channels for managing user allocations and other tasks.
Multiple antenna ports - Spatial Multiplexing and Transmit Diversity
The LTE downlink frame can be configured to use multiple antenna ports to transmit data to the UEs. Multiple antenna ports can be used to provide greater data reliability (transmit diversity) or to increase data rate (spatial multiplexing).
Transmit diversity uses multiple C-RS antenna ports on the base station to transmit the same amount of data as one antenna port. In this case, the data is coded so that the signals from the antenna ports add together in the channel allowing the UE to equalize and demodulate the data with greater reliability. In addition, the UE only needs one antenna to demodulate the data. Transmit diversity is not technically MIMO since MIMO stands for multiple inputs and multiple outputs.
Spatial multiplexing can be used to send data to UE's that have more than one receive antenna. Spatial Multiplexing uses multiple antenna ports to increase the data transmission capacity of the frame by using space as a third dimension (in addition to time and frequency) through which to send data. Spatial Multiplexing can be done using C-RS antenna ports (0-3) or UE-RS antenna ports (ports 7-8 for Dual-Layer Beamforming).
Modulation
Channels and signals are handled at different stages of the transmit chain. Channels contain data that originates at higher layers.
Signals, which include the reference signal and sync signals, contain known information that does not originate from higher layers. The signals are used by the receiver to perform certain Physical layer functions such as synchronization and equalization.
Signal subcarrier values are generated and mapped directly onto the OFDM subcarriers. Channel data undergoes several other steps before being mapped onto the OFDM subcarriers.
The following is an illustration of the LTE downlink transmit chain for a user's PDSCH channel. In this example, there are four C-RS antenna ports and three layers. The PDSCH channel shown below is using spatial multiplexing on the C-RS antenna ports. Other channels go through a similar process, except that they use one codeword and Transmit Diversity only.
Note that multi-layer PDSCH allocations using UE-RS beamforming do not undergo precoding.
The different sections of the illustration above are explained in the following sections.
Codewords
Codewords are simply separate streams of data that contain the information to be sent through a physical channel. There are two codewords defined for LTE: CW0 and CW1. Every channel uses CW0. PDSCH (user data) has the option of using CW1. Also, CW1 is only available when using spatial multiplexing.
The first step in the modulation process is to place data that is to be transmitted through a particular channel into the codewords for that channel.
Scrambling and modulation
Next, the data in each codeword is scrambled using a scrambling sequence for protection against burst errors. Then the binary data from this scrambling process is split into chunks depending on the modulation type chosen (6 bits for 64QAM, 4 bits for 16QAM, etc.) and mapped to complex-valued modulation symbols.
Codeword to layer mapping
Once a physical channel's codewords have been scrambled and modulated, the codewords are mapped onto the layers. There can be up to as many layers as there are antenna ports.
Codeword-to-layer mapping splits the data into layers. Since codeword-to-layer mapping is basically a demultiplexing process, the total number of modulation symbols in all layers remains the same as that in the codewords.
Below is a table that lists the different codeword-layer combinations defined in the standard for PDSCH transmitted on C-RS antenna ports:
At this point in the transmit chain for the current physical channel, the layer data is still specific to the physical channel. There is no concept of "frame" (resource blocks, subcarriers, antenna ports, or other channels and signals). Also, each physical channel can have a different number of layers.
The LTE demodulator provides traces that show IQ or error vector data vs. subcarrier or symbol. The content of these traces comes from the selected layer. However, layers do not exist in the context of resource blocks. How can these traces show layer data in the context of resource elements and symbols?
The answer is that the demodulator undoes the precoding to recover the original modulation symbols in the layers for each physical channel and then remaps those modulation symbols back onto the physical channel allocations in the frame. The values shown on the layer traces do not have a direct physical correspondence to the subcarrier that they are mapped to. However, there is still an indirect correspondence between modulation symbols and the actual subcarriers. For instance, if you corrupt one of the subcarriers in the physical channel's allocation by adding a sine wave at that particular frequency, you will see that the EVM of more than one modulation symbol on the physical channel's layer trace will be affected.
Precoding
Next, the layers are precoded using a precoding matrix defined by Section 6.3.4.2 in 3GPP TS 36.211. The result of precoding is a set of modulation symbols that are to be mapped directly onto the subcarriers. Precoding involves multiplying the layers matrix with a precoding matrix which creates the antenna port subcarrier values that are sent to the OFDMA mapper and then to the antenna ports.
For the single-antenna case, precoding is just a direct assignment of the layer contents to the antenna port data for the current physical channel.
For multiple-antennas, there are two types of precoding: transmit diversity precoding and spatial multiplexing precoding. In addition, spatial multiplexing can be either Without CDD (cyclic delay diversity) or with Large Delay CDD.
Spatial Multiplexing precoding reduces the correlation between the layers. This makes it easier for the antenna port signals to be separated using MIMO techniques once they are received.
Instead of precoding, beamforming can be used. Multi-layer spatial multiplexing can also be done using UE-RS antenna ports and each layer can be beamformed differently from the others.
OFDMA Mapping
After layer data for the physical-layer channels is precoded to create C-RS antenna port data, the OFDMA mapper combines the precoded values from physical-layer channels together with the reference signal and sync signals and places the subcarrier values into the appropriate locations in an OFDM symbol.
This OFDMA symbol mapping is performed separately for each antenna port. For more information about antenna ports and their respective contents, see the Antenna Ports and Transmit-Receive Paths topic.
OFDM Modulation
After values have been assigned for all subcarriers in an OFDM symbol for an antenna port (including the reference signal and control channels), the symbol is sent through an IFFT, which converts the symbol into time data. A cyclic prefix is appended and the time data is transmitted. The following image illustrates the entire TX chain for the single antenna case, which performs OFDM modulation directly after scrambling and modulation (no precoding). The difference when precoding is used is that the subcarrier values in the image below are replaced with the complex antenna port values that are a combination of the subcarrier values from each layer.
OFDM has a large peak-to-average power ratio which means that the amplifiers have to be of higher quality and are more expensive (and are also more power-hungry).
Synchronization
There are two downlink synchronization signals, the Primary Synchronization Signal (P-SS) and the Secondary Synchronization Signal (S-SS).
For FDD, P-SS is present in the last symbol, and S-SS is present in the second-to-last symbol of slots 0 and 10 in every frame.
For TDD, P-SS is present in the third symbol in slots 2 and 12 in every frame. S-SS is present in the last symbol of slots 1 and 11 in every frame.
The middle 72 subcarriers in these symbols are reserved for P-SS and S-SS, but only the center 62 subcarriers are used so that sync signals are more recognizable (easier to cross-correlate).
The location of these sync signals is the same for every bandwidth, which makes locking onto a signal easier when the bandwidth is not known.
Reference signals
The downlink LTE reference signals are discussed in the following sections.
Cell-specific Reference Signal (C-RS)
The Cell-specific Reference Signal (abbreviated C-RS or just RS) is transmitted on resource elements spread throughout the frame in specific locations as defined by the standard. The Cell-specific RS is used by the UE's to compensate the downlink frame for channel frequency response and cross-channel effects so that the signal can be demodulated.
Antenna ports 0-3 each have unique C-RS locations. Antenna ports do not transmit on resource elements allocated for RS on other antenna ports.
UE-specific Reference Signal (UE-RS)
In addition to the Cell-specific RS, the base station may transmit UE-specific RS in RBs allocated to the PDSCH of a UE. In LTE Release 8, UE-specific RS is used to accomplish single-layer beamforming and is transmitted on antenna port 5.
In Release 9, UE-RS for single-layer beamforming can be transmitted on antenna ports 5, 7, or 8. UE-RS for dual-layer (spatial multiplexing) beamforming is transmitted on antenna ports 7 and 8. See 3GPP TS 36.211, Section 6.10.3 for more information.
The LTE demodulator only supports viewing beam patterns for linear antenna arrays. The number of elements in an antenna group and the spacing between elements are specified by the Antenna Group parameters.
Positioning Reference Signal (P-RS)
The Positioning RS is used to enhance UE geolocation accuracy. The P-RS is transmitted periodically in certain frames and occupies certain resource elements within a rectangular area in the frame (RBs x SFs) as defined by the P-RS parameters.
Multicast/Broadcast Single Frequency Network Reference Signal (MBSFN-RS)
The MBSFN-RS is used to compensate for the downlink channel effects on the Physical Multicast Channel (PMCH), which contains the multicast/broadcast data, and is only transmitted during MBSFN subframes.
Physical channelsControl channels
Control channels provide the information needed to manage the transmission of data on the user channels and facilitate connecting to the base station. These channels are placed in specific locations in the frame.
The following table lists the control channels and a short description.
Shared channel
The physical downlink shared channel (PDSCH) contains the data being sent to users. All resource blocks are available for allocation, but only the subcarriers not reserved for control channels are available for carrying data.
Users are allocated rectangular areas of resource blocks and expect to find their data in those locations. Allocations can change each half-frame to work around channel effects such as frequency nulls.
Multicast channel
The Physical Multicast Channel (PMCH) supports the MBMS (Multimedia Broadcast/Multicast Service) and carries data that is intended for multiple users. A single cell (broadcast) or multiple cells (multicast) can participate in transmitting the data. The signals from each cell combine at the UE and provide an overall higher power.
MBMS signals are transmitted in Extended CP mode to mitigate the greater (than multipath) time difference of arrival between multiple cell transmissions due to the distance from each cell to the UE.
- |
- +1 赞 0
- 收藏
- 评论 0
本文由叫我大表哥吧转载自Keysight zhihu,原文标题为:LTE物理层概述 LTE Physical Layer Overview,本站所有转载文章系出于传递更多信息之目的,且明确注明来源,不希望被转载的媒体或个人可与我们联系,我们将立即进行删除处理。
相关研发服务和供应服务
相关推荐
Massive MIMO是什么意思?
Massive MIMO是第五代移动通信(5G)中提高系统容量和频谱利用率的关键技术,是大量天线的波束赋形。
技术探讨 发布时间 : 2024-06-15
什么是波束成形?
波束成形就是对波束的形状进行构造,波束成形、波束形成、波束成型和波束赋形意思相同。本文KEYSIGHT分析了Wi-Fi为什么要用波束赋形,并详细介绍了波束赋形的方法和波束赋形系統。
技术探讨 发布时间 : 2024-03-25
OFDM Introduction
This topic discusses the basic concepts of OFDM and how OFDM is implemented in 802.11a WLAN modulation. The basic OFDM principles will be introduced using a simple analog OFDM implementation and then those concepts will be extended to the digital domain with a simple digital OFDM implementation which utilizes theFFTtransform and DSP technology. The discussion ends with an explanation of how OFDM is implemented in 802.11a WLAN and how the OFDM symbol and burst is created.
技术探讨 发布时间 : 2024-01-16
解析射频识别技术 (RFID)是什么及RFID和条形码的区别
RFID射频识别技术可以建置于所有的自动资料撷取领域, 其主要由射频 (RF)来提供非接触式的物体识别。目前RFID射频识别技术应用领域从工业自动化、门禁管理、动物识别和电子护照, 到医疗、票务与库存追踪不等。如今RFID解决方案在大型企业的研发计画上备受瞩目。举例来说, 自动识别领域的成长, RFID射频识别技术功不可没,它提供了非接触式智慧卡、生产自动化和电子供应链所需的基本技术。
设计经验 发布时间 : 2023-12-05
仿真分析工作者们是如何做到从繁琐的优化工作中解脱出来的?
PathWave System Design(SystemVue)是Keysight公司的前沿软件,用于通信系统中射频架构的高级设计和仿真。PathWave System Design (SystemVue)允许用户从系统的角度构建基带和RF架构。它包括两个仿真引擎,允许对任意体系结构进行仿真。数据流模拟引擎完全支持数字系统。
设计经验 发布时间 : 2023-11-03
为什么太赫兹技术是6G关键技术之一?
太赫兹技术在光学领域有一个为大众所熟知的名字 — 远红外线。下一代通信系统中的无线通信频段向毫米波、太赫兹和可见光等更高频段发展,与传统感知频段将产生越来越多的重叠。根据预测,6G通信技术将实现每秒 1 TB 的下载速度、1 微秒的时延以及无限的带宽。 6G通信将赋能我们通过各种创新方式与周围环境进行交互,包括即时通信、互联机器人、自治系统以及无线人工智能交互等。
设计经验 发布时间 : 2023-12-12
详解实时频谱分析仪原理
所谓实时频谱分析仪(Real-Time Spectrum Analyzer - RTSA)就是指能实时显示信号在某一时刻的频率成分及相应幅度的分析仪,它能够帮助电子工程师完成频谱观测、功率测量以及复杂信号解调分析等工作。
设计经验 发布时间 : 2024-03-08
【经验】Keysight PathWave 89600 矢量信号分析软件进行矢量信号解调的原理和方法介绍
随着无线标准的发展,测量技术变得更加复杂,了解矢量信号解调的基本原理和方法变得越来越重要。凭借在数字信号处理、通信信号和矢量信号分析方面的扎实基础,Keysight PathWave 89600矢量信号分析软件在系统设计和研发方面发挥着巨大的作用。
设计经验 发布时间 : 2021-08-24
RFID Modulation Analysis (IoT Modulation Analysis) 89600 VSA Software
型号- 89601BN,89600B,89601C,89601B,89601200C,89600,89601BHTC,89601BK-BHC
PathWave Vector Signal Analysis (89600 VSA)
型号- 89604C,89601PSMC,89602C,89601BHQC,89601DVBC,89601CC1C,89600 VSA,89601AYAC,89601BHMC,89601B7RC,89601BHGC,89603C,89601CC2C,89601BHXC,89601CSDC,89601B7NC,89601BAXC,89601C,89601BHPC,89601BHTC,89601BHHC,89601BHNC,89601200C,89601101C,89601EVMC,89601301C,89601BHFC
解析频域和时域的关系
频域和时域分析是分析信号的基本方法,是从不同的角度来描述信号的特性。信号的特性可以在时域上和频率域上得到反映。本文是德科技解析了关于频域和时域的关系。
技术探讨 发布时间 : 2024-03-22
【经验】解析什么是实时频谱分析仪的频率模板触发和时间门触发
Keysight信号分析仪提供实时功能,可以连续处理40至160 MHz射频带宽内的所有样本。因此,它们可以无间隙地进行分析和触发,不会错过信号特性。实时分析作为信号分析仪的选件或更新提供。
设计经验 发布时间 : 2023-09-07
现货市场
服务
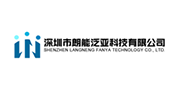
提供是德(Keysight),罗德(R&S)测试测量仪器租赁服务,包括网络分析仪、无线通讯综测仪、信号发生器、频谱分析仪、信号分析仪、电源等仪器租赁服务;租赁费用按月计算,租赁价格按仪器配置而定。
提交需求>
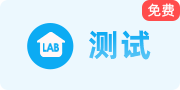
配备KEYSIGHT网络分析仪,可测量无线充电系统发射机/接收机线圈的阻抗,电感L、电阻R、电感C以及品质因数Q,仿真不同充电负载阻抗下的无线充电传输效率。支持到场/视频直播测试,资深专家全程指导。
实验室地址: 深圳 提交需求>
登录 | 立即注册
提交评论